[回上頁 | 顯示此文件為可列印格式]
此文件提供者: shuYing - [評分 : 0.00 (0 票選) | 評分!]
The Japanese Quail
Makoto MIZUTANI
Laboratory
Animal Research Station,Nippon Institute for Biological Science, Kobuchizawa,
Yamanashi, Japan, 408-0041
The
Japanese quail belongs to the order Galiformes,
genus Coturnix, and species japonica. The
scientific designation for Japanese quail is Coturnix
japonica, different from the common quail (Coturnix coturnix). The
Japanese quail is found in Japan, Korea, Eastern China, Mongolia and Sakhalin as
migrating birds.
The plumage
color of the wild type is predominantly dark cinnamon brown. However,
adult females have pale breast feathers that are speckled with dark colored
spots. Adult males have uniform
dark rust-red feathers on the breast and cheek (Fig. 1). Sex
differences in plumage color appear about 3 weeks of age but not at 0 – weeks of age.
The
eggshell color of the wild quail is white, flesh-tint, light brown or speckled
blue and/or brown. The size, shape and color pattern on the eggs vary
considerably among females. These differences in egg color have been proposed as a means
of identifying hens.
The
Japanese quail is only one of many animals domesticated by the Japanese. The first record of wild Japanese quail appeared in
the eighth century in Japan.
Thereafter,
several records of wild Japanese quail were found in several eras in Japan.
The Japanese quail was semi-domesticated during the sixteenth century as
a singing bird. Within the period from
1907 to 1941, the Japanese quail was selected for increased egg production. In 1941, about 2 million Japanese quail were kept. However, the majority of these domesticated quail
were lost during the Second World War. After
the war, the Japanese quail egg industry was rebuilt from the few remaining
domesticated birds, possibly with the addition of domesticated lines from Korea,
China, Taiwan and quails captured in the wild. Japanese quail are now farmed mainly for egg
production in Japan. In Europe, they are
selected for increased body weight for meat production. The body weight of meat quail is 2 – 3
times heavier than that of the egg production type.
Currently,
about seven million Japanese quail are kept in Japan. About 4 million birds are kept in Toyohashi City in
Aich prefecture. About 70 percent of the
eggs are produced in this area. Recently,
sex-linked brown plumage color has been used for auto-sexing in the quail
industry in Toyohashi.
As
mentioned above, the Japanese quail was used first as a singing bird and then as
an egg producer. It
later became popular as a laboratory animal, because of its small body size,
little consumption and rapid maturation.
Some
standard biological data are shown in Table 1.
In
Japan many Japanese quail strains were established and derived from commercial
birds.
Some of them are also registered in the
International Registry of Poultry Stocks (Somes, 1988). These
strains are classified into the following five groups with characteristics
described as follows:
I. Random bred closed colonies in which as many
varieties are maintained as possible.
I-1.
WE strain: A random-bred white eggshell strain with wild plumage color
was established in 1972 and maintained at the Nippon Institute for Biological
Science (NIBS).
I-2. WT
strain: A
random-bred
colored eggshell strain with wild type plumage color maintained at Hiroshima
University.
I-3. Commercial
strain: A
random-bred
colored eggshell strain with wild type plumage color maintained at the National
Institute of Livestock and Grassland Science.
I-4.
Wild strain: A random-bred colored eggshell strain with wild type plumage
color derived from wild Japanese quail captured at Mt. Fuji. This strain is maintained at the National Institute
of Livestock and Grassland Science.
I-5.
Normal strain: A random bred colored eggshell
strain with wild type plumage color maintained at Okayama University.
II. Closed
colonies in which some specific marker genes (plumage color, eggshell color,
blood typing et al.) are fixed.
II-1. PS
strain: A
random bred autosomal recessive pansy plumage strain maintained at Hiroshima
University (Tsudzuki and Wakasugi,
1987).
The pansy chicks show
light yellow down with three
narrow black stripes on the back. The
adult plumage is basically composed of three colors, i.e.
rust, black and white. Black is predominant on
the head. The male has a brown or
heavy rust face.
The
female shows a wheat-straw colored face with black stripes. In
the back feathers, color bands are arranged from the base to the tip as follows:
a gray base, mixed area of rust and black, large black area, a rust area and
white tip. The rachis color is
white at the base and becomes dark toward the tip.
II-2. YWE
strain:
Established in 1974. A random bred white
eggshell strain maintained at NIBS. Segregated
for autosomal homolethal yellow plumage color gene.
II-3. AMRP
strain: Established
in 1974 and maintained at NIBS by random breeding in a closed colony. Selected using natural agglutinin for mouse red
blood cells. This strain is
homozygous for blood group system with following loci by lectins: Sn/Sn (Glycine soja) (Mizutani et al., 1977a, b), Ns/Ns
(fruits bodies of Naematoloma sublaitum)
(Mizutani et al.,
1981). The genes of Es-Da
isozyme in red blood cells and autosomal recessive plumage color panda(s) of
this strain are homozygous too. The
Panda is a spotted plumage color mutant shown in Fig. 2 (Mizutani et al., 1974). The
chicks are primarily white, however, wild type down or feathers are sprinkled
around the eyes and ears and on the head, back, tail, secondary flight and
covert feathers.
II-4. SBPN
strain: Established
in 1973 by pedigree breeding at NIBS and maintained to date by random breeding
in a closed colony. This strain is
homozygous for blood group systems with the following loci by lectins: sn/sn (Glycine soja), ns/ns
(fruits bodies of Naematoloma sublatium),
and for the autosomal recessive plumage color panda gene(s).
II-5.
PNN strain: Established in 1972 and maintained at NIBS as a
random-bred closed colony with colored eggshells and wild type plumage. The strain is homozygous for blood group systems with Ly2/Ly2
locus by isoimmune serum (Katoh and
Wakasugi, 1981)
and the following loci by lectins: pn/pn
(Peanut) (Mizutani et al., 1977), Sn/Sn,
and ns/ns.
II-6. TKP
strain: Obtained
from the Takeda Co. in 1983 and maintained at NIBS as a random-bred closed
colony with colored eggshells and wild type plumage. This
strain is homozygous for blood group system with Ly3/Ly3
locus by isoimmune sera, following loci by lectins: Sn/Sn
and Ns/Ns, and for Es-Db
isozyme of red blood cells.
II-7.
GUC strain: Obtained from Gifu University in 1994 and
maintained at NIBS. The
strain is homozygous for autosomal recessive eggshell color celadon (ce) (Ito et al., 1993). The
eggshell color resembles
Sung era porcelain. A spectrophotometric
analysis revealed that celadon eggshell pigments are protoporphyrin and
biliverdin as in the wild type eggshell, but the protoporphyrin content is much
lower than that in the wild type. The biliverdin content
was 44% of the wild type.
A quail with both genes of eggshell color celadon and white
homozygously lays a pure white egg (Fig. 3).
III. Closed
colonies used as animal models for human hereditary diseases, malformations and
abnormalities controlled by one or few genes.
III-1.
S strain
Mutant genes: silver plumage, B, and the Amy-2A, are kept in the strain. Silver is controlled by an autosomal incomplete
dominant gene. Homozygous quail show
white plumage and a retinal defect (ring retina). A central part of the retina lacks pigment (Homma et al.,
1969). Grossly, a circular area
of hypopigmentation in the posterior retina with thinning in the subjacent
sclera is observed in all B/B
homozygous quails. As the birds mature, the
thinned sclera progresses to scleral ectasia. Posterior scleral ectasia may have developed secondarily to a
congenital structural defect in the posterior portion of the sclera associated
with general ocular defects. Heterozygous
quail show grayish plumage except for several white feathers on the wing tip. Recently, silver homozygous quail were reported to
have mutations in the Mitf gene. The
Mitf gene in B/B homozygous quail
impair osteoclastic bone resorption (Kawaguchi et
al., 2001).
The same mutant is kept at NIBS as a GUB strain
(Fig. 4).
III-2. DFND
(dark feather nervous disorder) strain
The mutant is controlled by an
autosomal recessive gene. The
plumage of chicks and adults is dark brown and the primary and secondary
feathers are frayed.
The
mutant is characterized by cerebellar functional disorders involving fine
tremors in the extremities and trunk, gait disturbance and tumbling when the
quail is active or excited. The mutant quail shows smaller cerebellum profiles
than the normal.
In
the mutant’s cerebellum, the Purkinje cell soma is small and fusiform in
shape, independent of its location. In the normal quail, the Purkinje soma is
flask-shaped. Purkinje
cells in the mutant are more intensely stained in the Bodian and Nissl
preparations. The mean cell number per unit area of Purkinje
cells and granular cells is not different from the normal quail.
This mutant is similar to the Reeler mouse in
histopathology and clinical features. However,
this mutant lacks Golgi II neurons in the white matter of the cerebellum as
observed in the Reeler mouse (Ueda et al.,
1979).
III-3. RWN strain
Established in 1976 and
maintained at NIBS. Glycogen storage disease
type II (acid maltase deficiency, AMD) appears in all quails in this strain. The AMD is controlled by an autosomal recessive
gene.
The AMD quail symptoms involve wing dysfunction
(Fig. 5). Histopathologically, the cells in the liver, heart
and skeletal muscles show cytoplasm with decreased staining by hematoxylin and
eosin. PAS-positive material
deposition is observed in the liver, heart, skeletal muscles and to a lesser
extent in the brain, intestine and gizzard. This
material is digested by diastase in all affected tissues and considered
glycogen. The superficial
pectralis muscle is most severely affected in the skeletal muscle. With 4-MUG as the substrate, the acid maltase
activity is decreased in the femoral muscle, superficial pectral muscle, heart
muscle and liver of affected quail. However,
there is no difference in the neutral maltase activity in those tissues
(Murakami et al.,
1982).
We attempted to establish an
early onset strain by mating quails that showed wing dysfunction at 3 weeks of
age. However, we occasionally found some birds with
normal behavior at 10 weeks of age in every generation. From original early onset AMD quails (RWNE strain),
we isolated late onset AMD quails (RWNL strain) with a normal wing functions in
spite of the presence of pathological changes in the pectral and wing muscle. So far, late onset AMD quails have not exhibited
the severe wing dysfunction found in early onset AMD quails even after 35 weeks
of age. The segregation ratio for the early onset type and
late onset type in the RWNE strain is 2:1. The
RWNL strain produces only the late onset type AMD quail. The
hatchability of the RWNE strain is always lower than that of the RWNL strain. These
results indicate that a dominant modifier gene influences the severity of AMD in
Japanese quail. Namely, quail that have
a dominant modifier gene homozygously die before hatching. The heterozygous quail show early onset type AMD. The recessively homozygous quail show late onset
type AMD. Histopathological analysis could not detect the
cause of death in quail embryos that had a homozygous dominant modifier gene. These
three
types of AMD quail resemble the human infantile form, child form and adult form,
respectively.
Enzyme replacement therapy for
AMD quail using human recombinant GAA showed a decrease in glycogen in the heart
and liver (Kikuchi et al., 1998).
In 1997, the full-length acid maltase cDNA of
Japanese quail was isolated from cDNA library derived from Japanese quail liver
(Kunita et al., 1997).
III-4. LWC strain
Myotonic dystrophy quail
appear in two third of the quail in this strain. The
mutant is controlled by an autosomal dominant homo lethal gene. The disorder is clinically apparent as early as 28
days of age. It is characterized by
generalized myotonia, muscle stiffness and muscle weakness. Affected birds are identified by their inability to
lift their wings vertically upward and by their inability to right themselves
when placed on their dorsum. This
symptom is very similar to that in AMD quail (Braga et al., 1995).
Electromyographic studies in
mutant quail show high-frequency repetitive discharges comparable to those in
myotonic runs. These
discharges persist after nerve resection. The distinctive
histopathlogic changes in the various muscles examined are ring fibers,
sarcoplasmic masses and internal migration in the sarcolemmal nuclei. A slight decrease in the size of type IIB muscle
fibers and a slight increase in the size of the type IIA fibers are observed in
the M. pectralis thoracicus of
affected quails (Fig. 6). The typical muscle
lesions and multi-system involvement, manifested by testicular degeneration and
atrophy in affected male quail specimens and bilateral lenticular cataracts in 6
of 13 affected quail, suggest the resemblance of this inherited muscular
disorder to myotonic dystrophy in humans. The
relationship between the development of muscle lesions and age, as well as
immunohistochemical and ultrastructural features in this mutant quail are
described in reference (Tanaka et al., 1996).
III-5. Quv strain
Neurofilament deficiency
appears in all quail in this strain. The
mutants show head or body quivering, or both. This
trait is characterized by neurofilament deficiency in the axons of the cervical
spinal cord and the optic and sciatic nerves, and named “hypotrophic
axonopathy”. This characteristics is controlled by an autosomal
recessive gene (Mizutani et al., 1992). This
is the first mutant suggesting compatibility without neurofilaments (NFs) in a
vertebrate. The existence of this mutant showed that NFs are
not necessary to the maintenance of life.
The mutant strain (Quv) was
successfully established. Electron
microscopically and immunohistochemically, neurofilaments are not detected in
the axons or neuronal cell bodies in Quv. Axons in Quv are composed mainly of
microtubules, which are increased in number in relation to the axonal size (Fig.
7). Gel
electrophoresis and Western blot analysis indicate that the low, middle and high
molecular mass neurofilament subunits are markedly deficient in the brain,
cervical spinal cord and sciatic nerve of Quv. Immunohistochemically, the Quv
spinal cord has no immunoreactive products corresponding to low molecular mass
NF. However, the middle and high molecular mass NF
antisera stained axons (Yamasaki et al., 1992).
We hypothesize that the NF
deficiency in Quv results from an alteration in the filament assembly caused by
defective expression of low molecular mass NF (NF-L).
When the NF-L gene sequences of normal and mutant
quail were compared, the NF-L gene in the mutant was found to have a nonsense
mutation at the deduced amino acid residue 114. This
indicates that the mutant is incapable of producing even a trace amount of NF-L
protein in any situation. The
morphological features of the myelinated fibers in Quv were also studied in
detail (Zaho et al., 1995).
Furthermore, the neurotoxic effects of
acrylamide (Takahashi et al.,
1995),
β-β’-iminodipropionitrile
(Mitsuishi et al., 1993)
and 2,5-Hexanedione (Hirai et al.,
1999) were also investigated
using the Quv strain.
III-6. ET strain
This strain was found in the B
strain maintained at Nagoya University, and is now maintained at Hiroshima
University. Ear tufts and irregular
shaped ear openings appear in all quail in this strain. The mutant ear opening is oval shaped with a
fissure on its ventral margin.
Ear
tufts project from the ventral end of this fissure or the posterior margin of
the ear opening. The ear tufts are composed of a feathered peduncle.
The size of the ear tufts and the ear-opening
abnormality are variable.
In
5-day old ET strain embryos, the incidence of an incomplete closure of the
hyomandibular furrow is 91%. This
hyomandibular furrow abnormality seems to be the primary defect leading to the
ear opening and ear-tuft traits. This characteristics is controlled by an autosomal
recessive gene (Tsudzuki and Wakasugi, 1988).
III-7. TT
strain
In 1985, two chicks exhibiting
throat-tufts (TT) were found in the Japan-Taiwan mixture (JTM) strain maintained
at Nagoya University. The
TT strain is now maintained at Hiroshima University.
The throat-tuft phenotype in
Japanese quail is characterized by a throat tuft consisting of an epidermal
peduncle and covering feathers. Sometimes it is
accompanied by abnormal ear openings showing a wide cleft at the ventral margin
and, in some instances, an extra bony projection on the peduncle or in its
vicinity (Fig. 8).
The
incidence of throat tufts and ear opening abnormalities in 15-day embryos in the
TT strain are 50% and 17%, respectively. The
5-day TT embryos exhibited peduncle formation and hyomandibular furrow defects
that are thought to cause throat tuft and ear opening abnormalities,
respectively. Mating experiments suggest that the throat tuft
characteristic is controlled by an autosomal recessive gene that is allelic and
dominant to the ear tuft gene (Tsudzuki and Wakasugi,
1989, 1990).
III-8. HMM strain
Hereditary multiple
malformation (HMM), a new mutation in Japanese quail (Fig. 9), was discovered
among the progeny from a pair from the WT strain kept at Osaka Prefecture
University, now maintained at Hiroshima University.
This characteristics
is controlled by an autosomal recessive gene. The
majority of the homozygotes die on the sixth day of incubation and the
remaining die at various stages until 15 days of incubation. The homozygotes surviving to the late embryonic
stages have greatly shortened lower and upper beaks that are set apart and show
an early embryo-like body shape, with feather buds but no plumules. Furthermore, they show syndactylous polydactyly in
both the fore and hind limbs. In the abdomen of the
homozygotes, a portion of the ventriculus, liver and small intestine protrudes
out of the umbilicus region. In the skeleton of the
late HMM embryos, ossification is generally delayed and morphogenetic
abnormalities are observed all over the body. This
mutant has become a useful animal model in the morphogenesis research field (Tsudzuki et al.,
1998).
III-9. SLB
strain
Abnormal embryos with a short
lower beak (SLB) were discovered among the progeny from a pair from the DDL
strain maintained at Osaka Prefecture University, now maintained at Hiroshima
University.
The SLB individuals are
characterized by a shortened lower beak, opened mouth and small body size. These mutants die in the late embryonic stages. Skeletal analyses revealed that the Meckel’s
cartilage of the mandible is abnormally bent downward in its proximal portion. The
mandibular bones are formed around the abnormal Meckel’s cartilage, which
seems to be responsible for the shortened lower beak and the opened mouth. The
appendicular bones in the mutant embryos are also shortened.
Skeletal abnormalities are also observed in the
hyoid apparatus, ribs and cervical vertebrae. Genetic analyses revealed that the SLB mutation is
controlled by two linked autosomal recessive genes with a recombination
rate of 33%. This mutant might be a
useful animal model in the study of morphogenesis of the mandible, hyoid
apparatus, cervical vertebrae, ribs and appendicular bones (Nakane and Tsudzuki,
1998a; Nakane,
1998).
III-10. ZN strain
Abnormal embryos (Zazen)
showing crossed legs and opened eyes were discovered among the progeny from a
pair from the SBK strain maintained at Osaka Prefecture University, now
maintained at Hiroshima University. The
name of the mutant,
Zazen, is based on a leg condition similar to the religious mediation figure (=za-zen)
in the Zen sect. Zazen birds are
characterized by crossed legs, opened eyes and abnormal beaks. In this skeletal system, all mutant embryos have a
full-length fibula in both legs from the knee to the hock joint. The
femur is articulated with the fibula, but not the tibia.
Other
skeletal abnormalities are observed in the premaxilla, parietal, and ribs. All
Zazen mutants die in the late embryonic stages. Genetic analyses reveal that the
mutation is
controlled by two genes,
autosomal
dominant gene
and sex-linked
recessive gene. The latter is epistatic
to the former. This mutant might be a
useful animal model in the study of developmental leg skeleton aspects in
relation to the genes controlling morphogenesis (Nakane and
Tsudzuki,
1998b; Nakane,
1998).
IV. Closed
colonies that were established by successive selections of specific
characteristics controlled by many genes.
IV-1. LAP
strain
Hyperlipidemia
atherosclerosis-prone (LAP) strain susceptible to experimental atherosclerosis
was established by repeated breeding of highly susceptible quails induced by a
diet containing 1% cholesterol. The LAP strain was
derived from the HAP strain established by Ohtsuka Pharmaceutical Co. Ltd. The following findings are described.
(1)
The retarded rate of cholesterol biosynthesis or
catabolism is not responsible for hypercholesterolemia (Nagata et
al.,
1996).
(2)
Cholesterol feeding had no effect on the level of cholesterol, chemical
composition or fatty-acid composition of the high-density lipoprotein fractions
in LAP and normal strain.
Although the lipoprotein and apoprotein profiles in LAP quail
show resemblance to those of normal quail, expression of the 470 kDa protein in
the lipoprotein appears to be pronounced in the LAP quail (Nagata et al.,
1997).
(3)
The tissue distribution for apolipoprotein A-I (apo A-I) is similar in
both LAP and normal strains, but LAP quail expresses more apo A-I-mRNA than
normal quail in all tissues examined (Iwasaki et al.,
1999).
(4)
The DNA sequence of LAP apo A-I cDNA is similar to that in the normal
quail (Iwasaki et al.,
1999).
(5)
The structure and expression of the major low molecular weight apoprotein
has no relevance to higher LAP susceptibility to experimental atherosclerosis
(Iwasaki et al.,
2000).
IV-2. H2 and L2 strains
The High responder strain (H2)
and low responder strain (L2) were established by selective breeding experiments
through 50 generations for high and low responders to inactivated Newcastle
disease virus (NDV) antigen at National Institute for Environmental Science
(NIES) (Takahashi et al.,
1984). Hemagglutination inhibition titers of the H2 strain
and L2 strain in F18 are 8.54log2 and 1.85log2, respectively. The mortality of the H2 strain after NDV challenge
was lower than that for the L2 strain. The H2
strain is a high responder when they are immunized with influenza virus, sheep
red blood cells and S. pullorum. There is no correlation between antibody production
and reproduction. The mitogenic response
of lymphocytes to PHA is higher in the H2 strain than the L2 strain. The rate of spontaneous rosette cells in blood
lymphocytes with either rabbit or fowl erythrocytes in the H2 strain were higher
than that in the L2 strain (Inooka et al.,
1984).
IV-3. LL,
SS, RR and SL strains
Three strains for either large
(LL) or small (SS) body size at 6 weeks of age and random-bred
control strain (RR) were developed by successive selection of 80 generations at
Saga University. At 12 weeks of age, the
body weights of LL, SS and RR strain are 212.0 g, 66.5 g and 104.8 g,
respectively.
Researches using above strains are as follows:
(1)
Comparative studies on body weight, tibia length
and abdominal weight (Ardiningsasi et al.,
1992).
(2)
Investigation of the feed conversion to body weight gain and egg
production (Okamoto et al.,
1989).
(3)
Genetic studies on muscle protein turnover rate, acid protease activity
and calcium-activated neutral protease activity (Maeda et
al.,
1986; Maeda et al.,
1989;
Maeda et al.,
1991).
(4)
Research on the heterosis effects existing in the productive traits
(weekly body weight, egg laying traits, fertility, hatchability, rate of raising
and viability) (Piao et al.,
2002).
The SL strain was derived from
the SS strain by selecting large body size.
The
SS and SL strains would be useful for research on body size miniaturization from
molecular biologic and metabolic aspects to provide an insight into the
evolutionary mechanism (Suda et al.,
2002).
V. Closed
colonies that are used in auto-sexing of newly hatched chicks.
V-1. AWE strain
This strain was established in
1975 at NIBS, and is a random-bred white eggshell strain with a homozygous
sex-linked albino gene. As the AWE strain was
previously crossed with the WE strain; the genetic background of these two
strains is similar. These strains are used
to investigate the screening and testing of endocrine disruptors on the gonads
of Japanese quail.
It
is possible to check the sex of chicks and embryos using their plumage color
when a male AWE quail is mated with female WE quail (Fig. 10).
The strain was established at
NIBS and is a random-bred white eggshell strain segregated for sex-linked brown,
sex-linked cinnamon and autosomal yellow plumage color genes.
V-3. SBC strain
The strain was established at
Hiroshima University. The sex-linked brown and
the sex-linked cinnamon genes are segregated.
Acknowledgements
The
author thanks Dr. K. Yamanouchi for reading the manuscript.
References
Ardiningsasi,
S. M., Maeda, Y., Okamoto, S., Okamoto, S. and Hashiguchi, T. 1992. Comparative studies of body weight, tibia length
and abdominal fat weight among lines selected for body size in Japanese quail, Coturnix
coturnix japonica. Jpn. Poult. Sci.
29:
310-315.
Braga,
III, I. S., Tanaka, S., Kimura, T., Itakura, C. and Mizutani, M. 1995. Inherited muscular disorder in mutant Japanese
quail (Coturnix coturnix japonica):
relationship between the development of muscle lesions and age. J. Com. Path.
113: 131-143.
Hirai,
T., Mizutani, M., Kimura, T., Ochiai, K., Uemura, T. and Itakura, C. 1999. Neurotoxic effects of 2,5-hexanedione on normal and
neurofilament-deficient quail. Toxicologic Patholgy 27: 348-353.
Homma,
K., Jinno, M. and Kito, J. 1969. Studies
on silver-feathered Japanese quail. Jpn. J. Zootech. Sci. 40: 129-130.
Inooka,
S., Takahashi, S., Takahashi, H. and Mizuma, Y. 1984. Immunological traits in generations 7 to 12 of two
lines of Japanese quail selected for high or low antibody response to Newcastle
disease virus. Poultry Sci. 63: 1298-1302.
Ito,
S., Tsudzuki, M., Komori, M. and Mizutani, M. 1993.
Celadon: An eggshell color mutation in Japanese
quail. J. Hered. 84:
145-147.
Iwasaki,
H., Oku, H., Toda, T., Nasu, T., Miyagi, T. and Chinen, I. 1999. Apolipoprotein A–I of hyperlipidemia
atherosclerosis-prone (LAP) quail: cDNA sequence and tissue expression. Biosci.
Biotechnol. Biochem. 63: 29-34.
Iwasaki,
H., Oku, H., Toda, T., Nasu, T., Miyagi, T. and Chinen, I. 2000. The major low molecular weight apolipoprotein from
normal and hyperlipidemia atherosclerosis-prone (LAP) Japanese quail. Biochimica
et Biophysica Acta 1483: 316-324.
Katoh,
H. and Wakasugi, N. 1981. Studies on the blood
groups in the Japanese quail. Detection of three antigens and their inheritance.
Immunogenetics 13: 109-114.
Kawaguchi,
N., Ono, T., Mochii, M. and Noda, M. 2001. Spontaneous mutation in Mitf gene causes
osteopetrosis in silver homozygote quail. Dev. Dyn. 220: 133-140.
Kikuchi,
T., Yang, H. W., Pennybacker, M., Ichihara, N., Mizutani, M., Hove, J. L. K. and
Chen, Y. T. 1998.
Clinical
and metabolic correction of Pompe disease by enzyme therapy in acid
maltase-deficient quail. J. Clin. Invest. 101: 827-833.
Kunita,
R., Nakabayashi, O., Wu, J. Y., Hagiwara, Y., Mizutani, M., Pennybacker, M.,
Chen, Y. T. and Kikuchi, T. 1997. Molecular
cloning of acid α-glucosidase cDNA of
Japanese quail (Coturnix coturnix japonica)
and the lack of its mRNA in acid maltase deficient quails. Biochimica et
Biophysica Acta 1362: 269-278.
Maeda,
Y., Fujii, M., Okamoto, S. and Hashiguchi, T. 1989. Variation among lines selected for body size in the
fractional rate of degradation of protein and acid protease activity in the
muscle of quail (Coturnix coturnix
japonica). Biochemical Genetics 27: 603-611.
Maeda, Y., Hayashi, K.,
Hashiguchi, T. and Okamoto, S. 1986. Genetic
studies on the muscle protein turnover rate of Coturnix
quail. Biochemical Genetics 24: 207-216.
Maeda,
Y., Kawabe, K., Okamoto, S., Okamoto, S. and Hashiguchi, T. 1991. Genetical studies on muscle protein turnover rate
and calcium activated neutral protease activity in the skeletal muscle of the
Japanese quail (Coturnix coturnix japonica).
Anim. Sci. Technol. (Jpn), 62: 813-821.
Mitsuishi,
K., Takahashi, A., Mizutani, M., Ochiai, K. and Itakura, C. 1993. β,β’ iminodipropionitrile toxicity in normal and
congenitally neurofilament deficient Japanese quails. Acta Neuropathol. 86:
578-581.
Mizutani,
M., Chino, K., Umezawa, H. and Kuramasu, S. 1974. Genetic analysis of a new plumage panda in Japanese
quail (in Japanese with English summary). Exp. Anim. 23: 59-61.
Mizutani,
M., Nunoya, T., Yamasaki, H. and Itakura C. 1992. The hypotrophic axonopathy mutant in Japanese
quail. J. Hered. 83: 234-235.
Mizutani, M., Saito, T.,
Umezawa, H. and Kuramasu, S. 1981. A
new agglutinogen detected by Naematoloma
sublatium PHA in Japanese quail. ABRI(Animal Blood Group Research
Information) 9: 43-45.
Mizutani,
M., Umezawa, H. and Kuramasu. S. 1977a. Studies of the red blood cell agglutinogen in
Japanese quail detected by soybean PHA. Jpn. J. Zootech. Sci. 48: 227-234.
Mizutani,
M., Umezawa, H. and Kuramasu, S. 1977b. Studies
on the hemagglutinogen in Japanese quail detected by peanut PHA. Jpn. J.
Zootech. Sci. 48: 463-467.
Murakami,
H., Takagi, A., Nonaka, I., Ishiura, S., Sugita, H. and
Mizutani, M. 1982. Type 2 glycogen storage
disease in Japanese quail. In
Muscular
Dystrophy (Edited by S. Ebashi), Tokyo Univ. pp.
37-48.
Nagata,
J.,Maeda, G., Oku, H., Toda, T. and Chinen, I. 1997. Lipoprotein and apoprotein profiles of
hyperlipidemic atherosclerosis-prone Japanese quail. J. Nutr. Sci. Vitaminol.
43: 47-57.
Nagata,
J., Oku, H., Toda, T. and Chinen, I. 1996. Effect of dietary choresterol on the activities of
key enzymes of choresterol metabolism in hyperlipidemia and
atherosclerosis-prone Japanese quail. J. Nutr. Sci. Vitaminol. 42: 287-300.
Nakane,
Y. 1998.
Exploitation
of new animal models in Japanese quail (Coturnix
coturnix). Establishment
of embryonic skeletogenesis stages and analyses for newly discovered
morphogentic mutations. Doctorial
thesis, Osaka Prefecture University.
Nakane,
Y. and Tsudzuki, M. 1998a.
Morphological and genetic studies for new mutant of
Japanese quail. In: Proceedings of the 6th Asian Pacific Poultry
Congress (Nagoya, Japan), pp. 242-243.
Nakane,
Y. and Tsudzuki, M. 1998b.
A new morphological lethal mutation showing eye,
beak, and leg abnormalities in Japanese quail. In: Proceedings of the 8th
World Conference on Animal Production (Soul, Korea), Vol. II, pp. 148-149.
Okamoto, S., Kobayashi, S. and
Matsuo, T. 1989. Feed
conversion to body weight gain and egg production in large and small Japanese
quail lines selected for 6-week body weight. Jpn. Poult. Sci. 26: 227-234.
Piao,
J., Okamoto, S., Kobayashi, S., Wada, Y. and Maeda, Y. 2002. Study of heterosis effects on production traits of
Japanese quails. 2) Heterosis effects on the crosses between small line and
random bred population. Jpn Poult. Sci. 39: 139-146.
Somes,
R. G. Jr. 1988. International
Registry of Poultry Stocks, Storrs Agricultural Experiment Station, The
University of Connecticut, Storrs, Connecticut.
Suda,
Y., Imakawa, K. and Okamoto, S. 2002. Long
term selection for small body weight in Japanese quail. I: direct selection
response from 60 to 65th generation. Jpn. Poult. Sci. 39: 274-284.
Takahashi,
S., Inooka, S. and Mizuma, Y. 1984. Selective
breeding for high and low antibody responses to inactivated Newcastle disease
virus in Japanese quails. Poultry Sci. 63: 595-599.
Takahashi,
A., Mizutani, M.
and
Itakura, C. 1995. Acrylamide-induced peripheral neuropathy in normal
and neurofilament-deficient Japanese quails. Acta Neuropathol. 89:
17-22.
Tanaka,
S., Braga, III, I. S., Kimura, T., Ochiai, K., Itakura, C. and Mizutani, M.
1996. Inherited muscular disorder in mutant Japanese
quail (Coturnix coturnix japonica): an
ultrastructural study. J. Com. Path. 114: 325-337.
Tsudzuki,
M., Nakane, Y. and Wada, A. 1998. Hereditary
multiple malformation in Japanese quail: a possible powerful animal model for
morphogenetic studies. J. Hered. 89: 24-31.
Tsudzuki,
M. and Wakasugi, N. 1987. “Pansy”:
a plumage color mutant in Japanese quail. Jpn. Poult. Sci. 24: 327-335
Tsudzuki,
M. and Wakasugi, N. 1988. A
genetic defect in hyomandibular furrow closure in the Japanese quail: The causes
for ear-opening abnormality and formation of ear tuft. J. Hered. 79: 160-164.
Tsudzuki,
M. and Wakasugi, N. 1989. Throat
tuft: A new discovered morphological mutation in Japanese quail. J. Hered. 80:
433-441.
Tsudzuki,
M. and Wakasugi, N. 1990. A
genetic analysis on the throat-tuft character of Japanese quail (Coturnix coturnix japonica) based on the head-skeleton abnormality.
Jpn. Poult. Sci. 27: 393-397.
Ueda,
S., Ito, H., Masai, H. and Kawahara, T. 1979. Abnormal
organization of the cerebellar cortex in the mutant Japanese quail, Coturnix coturnix japonica.
Brain Research 177: 183-188.
Yamasaki,
H., Bennett, G. S., Itakura, C. and Mizutani, M. 1992.
Defective
expression of neurofilament protein subunits in hereditary hypotrophic
axonopathy of quail. Lab. Invest. 66: 734-743.
Zaho, J. X., Ohnishi, A.,
Itakura, C., Mizutani, M., Yamamoto, T., Hojo, T. and Murai, Y. 1995. Smaller
axon and unaltered numbers of microtubules per axon in relation to number of
myelin lamellae of myelinated fibers in the mutant quail deficient in
neurofilaments. Acta Neuropathol. 89: 305-312.
Fig.
1. Japanese quail (Left: female,
Right:
male)
Fig.
2. Japanese quail with panda plumage
Fig. 3. Egg
shell color (we: white, ce: celadon, pw: pure white)
Fig.
4. GUB strain (Silver plumage)
Fig.
5. AMD quail
Fig.
6. M.
pectoralis thoracicus of normal (left) and LWC (right) quail
Left:
Type αW (IIB) and αR
(IIA) fibers are well differentiated
Right: Note
a slight decrease in the size of the type αW
fibers with a relative
increase
in the size of type αR
fibers compared to the normal quail
Fig.
7. Electron microscopy of nerve
fiber of normal (left) and Quv (right) quail
Left:
axonal cytoskeleton of normal quail comprise a number of NFs with less
microtubules
Right:
NF central cores were not observed but microtubules were increased in number
Fig. 8. Dotted
white quail with a large throat tuft on the left side only (Photo
by M. Tsudzuki)
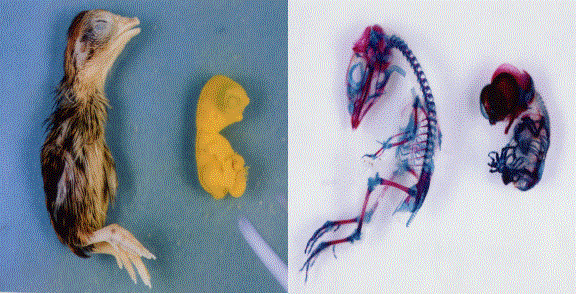
Fig. 9. Comparison
of an external appearance and skeleton of 13-days embryo of normal (left) and
HMM mutant quail (right) (Photo by M. Tsudzuki)
Fig. 10. Left:
Male of AWE strain, Right: Female of WE strain
[回上頁 | 顯示此文件為可列印格式]
此文件提供者: shuYing - [評分 : 0.00 (0 票選) | 評分!]